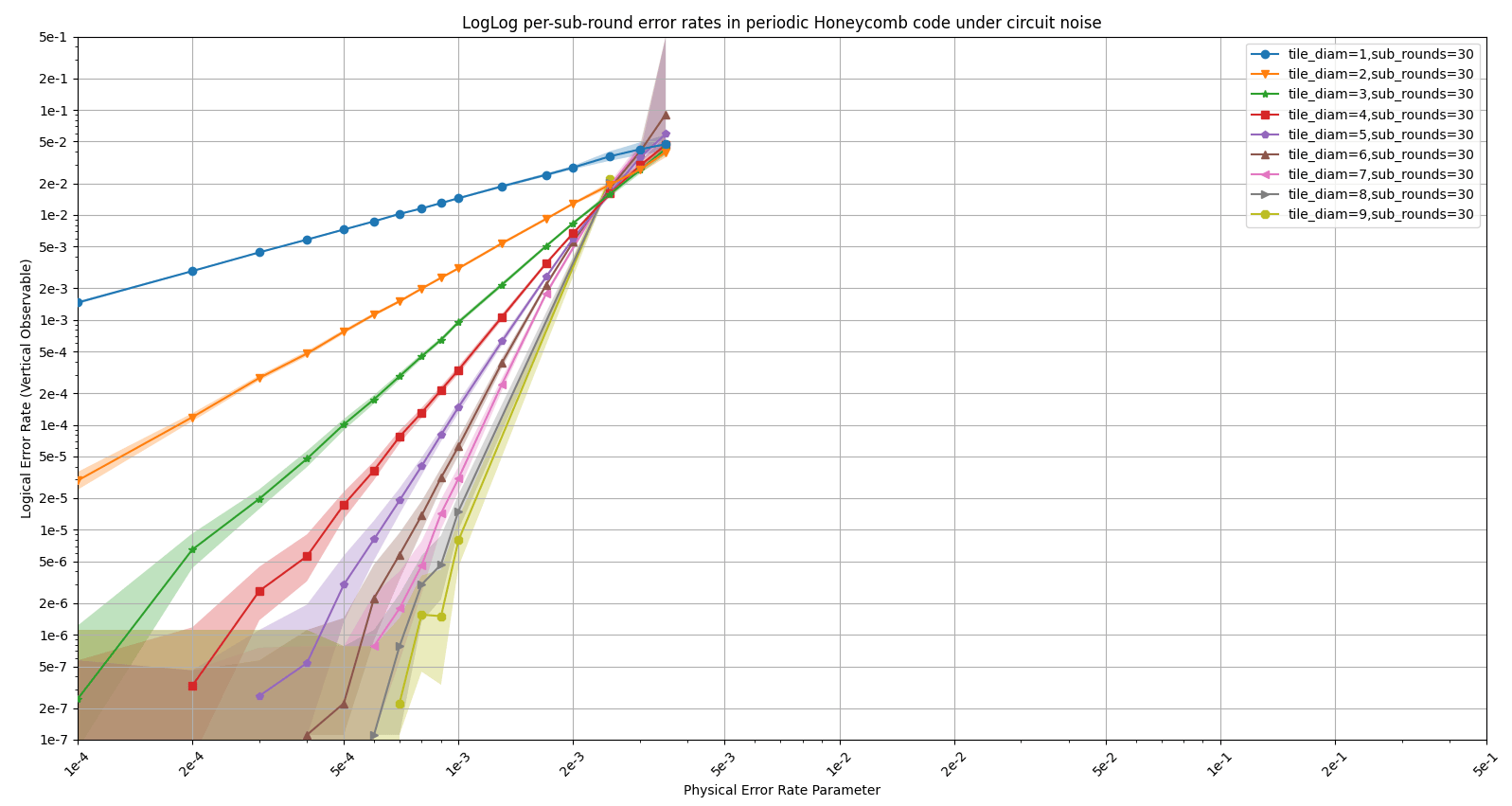
In the exciting world of quantum computing, there have been significant advancements in dynamically generated logical qubits.
These breakthroughs are revolutionizing the way we think about information processing and hold immense potential for the future.
Let’s dive into the fascinating realm of dynamically generated logical qubits and explore the cutting-edge developments in this field!
Imagine a superpowered computer that can solve complex problems with astonishing speed and efficiency. That’s precisely what researchers and scientists are striving for with dynamically generated logical qubits. These advancements take us beyond traditional binary computing and into the realm of quantum mechanics.
By harnessing the properties of quantum superposition and entanglement, logical qubits are paving the way for a new era of computing power.
So, what exactly are logical qubits, and how are they dynamically generated? In a nutshell, a qubit is the fundamental building block of quantum computing, analogous to a classical bit in traditional computing.
However, logical qubits are more robust and resistant to errors, making them ideal for quantum computation. They are generated by implementing specialized algorithms and control mechanisms that can effectively manage and manipulate quantum information.
Stay tuned as we delve deeper into the intricacies of dynamically generated logical qubits and uncover the latest advancements in this fascinating field. Get ready to be amazed by the potential of this groundbreaking technology!
Advancements in Dynamically Generated Logical Qubits
Quantum computing is a rapidly evolving field with enormous potential for revolutionizing various sectors, from cryptography to drug discovery.
One crucial aspect of quantum computing is the development of logical qubits, which are more robust and reliable than physical qubits. In recent years, there have been significant advancements in dynamically generated logical qubits, paving the way for more practical and scalable quantum computers.
This article delves into the latest developments in this exciting area of research and explores their implications for the future of quantum computing.
Role of Dynamically Generated Logical Qubits in Quantum Computing
Before diving into the advancements, let’s understand the role of dynamically generated logical qubits in quantum computing. Logical qubits are encoded in multiple physical qubits to create an error-tolerant and fault-tolerant qubit that can withstand noise and errors.
Dynamically generating logical qubits involves actively manipulating the physical qubits to correct errors and maintain the coherence required for quantum computations.
This approach has several advantages, including the ability to detect and correct errors in real time, making quantum computers more reliable and efficient.
Advancement 1: Error Correction using Surface Codes
One of the remarkable advancements in dynamically generated logical qubits is the use of surface codes for error correction. Surface codes are a type of error-correcting code that can detect and correct errors reliably.
They utilize a two-dimensional lattice of physical qubits, with each qubit interacting with its neighboring qubits. By measuring certain quantities and applying correction operations, errors can be detected and rectified.
Surface codes have shown promising results in improving the fidelity of logical qubits, making them less susceptible to errors caused by environmental factors or hardware imperfections.
Researchers have developed innovative techniques for implementing surface codes in quantum hardware, such as superconducting circuits or trapped ions.
These advancements have led to substantial improvements in the error rates of logical qubits, bringing us closer to building fault-tolerant quantum computers that can perform complex computations with high accuracy.
To gain a better understanding of the power of surface codes, consider the analogy of a crossword puzzle. Each physical qubit represents a square in the puzzle, and the connections between qubits create a network that allows errors to be detected.
Similar to how filling in certain squares can reveal errors in a crossword puzzle, measuring the properties of the physical qubits can uncover errors in quantum computations.
Surface codes provide a robust framework for detecting and correcting these errors, paving the way for more reliable quantum computers.
Advancement 2: Dynamical Decoupling Techniques
To combat noise and environmental fluctuations, researchers have been investigating dynamical decoupling techniques for dynamically generated logical qubits.
These techniques involve applying carefully timed pulses or sequences of operations to the physical qubits to counteract the effects of noise. By periodically flipping the qubits or manipulating their interactions, the detrimental effects of noise can be suppressed, leading to improved coherence and error rates.
One such technique is known as pulse-shaping, where tailored electromagnetic pulses are used to manipulate the quantum states of the physical qubits.
By carefully designing the pulse shapes and timings, researchers can mitigate the impact of noise and enhance the stability of logical qubits. The development of these dynamical decoupling techniques has opened up new avenues for improving the resilience and reliability of quantum computers, making them more practical for real-world applications.
Imagine trying to balance a pencil on its tip. The slightest disturbance can cause the pencil to fall. Similarly, quantum systems are incredibly sensitive to disturbances from the environment.
Dynamical decoupling techniques act as a virtual hand, continuously adjusting and stabilizing the physical qubits to prevent them from succumbing to noise. These techniques are vital for maintaining the delicate quantum states required for quantum computations.
Advancement 3: Hybrid Logical-Qubit Architectures
In pursuit of scalable quantum computers, researchers are exploring hybrid architectures for dynamically generated logical qubits.
These architectures combine different physical platforms, such as superconducting qubits, trapped ions, or topological qubits, to take advantage of the strengths of each platform while mitigating their individual weaknesses. By integrating multiple technologies, hybrid architectures offer greater flexibility, scalability, and error-correction capabilities.
One hybrid architecture gaining attention is the combination of superconducting qubits and topological qubits. Superconducting qubits are known for their fast gate operations, while topological qubits offer robust error correction properties.
By leveraging the strengths of both, researchers aim to create logical qubits that are both fast and resilient to errors. These hybrid architectures present exciting possibilities for building practical quantum computers that can outperform their individual components.
Think of hybrid logical-qubit architectures as a fusion of different musical instruments in an orchestra. Each instrument has its unique capabilities and limitations, but when combined, they create a harmonious and powerful sound.
Similarly, hybrid architectures bring together diverse quantum technologies, leveraging their strengths to overcome limitations and create more powerful logical qubits.
Advancement 4: Real-Time Error Monitoring and Mitigation
A crucial aspect of dynamically generated logical qubits is the ability to monitor errors in real time and apply immediate corrective measures.
With advancements in measurement techniques and control systems, researchers have made significant progress in developing methods for real-time error monitoring and mitigation.
By continuously monitoring the state of the physical qubits, researchers can detect errors as they occur and dynamically adjust the operations to correct or mitigate their effects.
Real-time error monitoring relies on sophisticated measurement protocols, such as quantum non-demolition measurements, which allow for precise and non-destructive state monitoring.
Combined with fast and efficient control systems, researchers can design feedback loops that automatically adjust the quantum operations in response to errors.
This real-time error detection and mitigation approach enables dynamic adaptation to the changing quantum system, ensuring the logical qubits remain in the desired state throughout the computation.
Imagine driving a car with a responsive autopilot system. As soon as the car starts veering off course, the system kicks in and makes the necessary adjustments to bring it back on track.
Similarly, real-time error monitoring and mitigation act as a quantum autopilot, keeping the logical qubits on the right trajectory and ensuring accurate and reliable quantum computations.
Advancement 5: Quantum Error Suppression Techniques
Quantum error suppression techniques are aimed at reducing the impact of errors on dynamically generated logical qubits without resorting to full-blown error correction.
These techniques exploit the redundancy of quantum information encoded in multiple physical qubits to suppress errors and enhance the reliability of quantum computations. By optimizing the encoding and decoding processes, researchers have developed innovative error-suppression protocols.
One such technique is the so-called concatenated encoding, where additional encoding levels are introduced to provide more layers of error suppression.
By cascading multiple levels of encoding, errors that escape one level can still be suppressed at subsequent levels, leading to improved fidelity and resilience of the logical qubits. While these techniques may not offer the same level of error correction as surface codes, they can significantly reduce the error rates, making quantum computations more reliable.
Consider building a sandcastle with multiple layers. Even if the outer layer is eroded by wind or waves, the inner layers remain intact and provide protection.
Quantum error suppression techniques work in a similar fashion, using multiple layers of encoding to safeguard the quantum information against errors. These techniques offer a balance between error correction and practicality, making them valuable tools in the realm of dynamically generated logical qubits.
Advancement 6: Topological Quantum Memory
Topological quantum memory is a groundbreaking advancement in dynamically generated logical qubits. It allows for the storage and manipulation of quantum information in a robust and highly coherent manner.
Topological qubits are characterized by their topological properties, which make them resilient to local noise and errors. By encoding quantum information in these topological properties, researchers can create logical qubits that are more stable and less prone to decoherence.
The development of topological quantum memory has been driven by the field of topological quantum computing, which explores the use of exotic particles, such as anyons, to perform quantum operations.
While the practical implementation of topological qubits is still a challenge, researchers have made significant progress in understanding and controlling these topological properties.
The potential of topological quantum memory lies in its ability to store quantum information for extended periods, providing a crucial building block for future quantum computers.
Imagine a shelf that can hold fragile objects, preventing them from breaking even if they are accidentally bumped.
Topological quantum memory serves as a similar protective shelf for quantum information. It shields the fragile quantum states from external disturbances, preserving their coherence and integrity.
Advancement 7: Fault-Tolerant Operations and Gate Implementations
Achieving fault-tolerant operations is an essential milestone in the development of dynamically generated logical qubits.
Fault-tolerant operations refer to operations or gates that can be applied to logical qubits without introducing errors or compromising their coherence.
Researchers are actively working on improving gate implementations to minimize error rates and increase the fidelity of logical qubit operations.
One approach to fault-tolerant gate implementations is the use of magic states, which are non-classical states that enable more efficient computations.
By carefully designing the interactions between physical qubits and these magic states, researchers can enhance the gate fidelity and reduce the errors caused by imperfect operations.
This advancement is crucial for achieving larger-scale quantum computations, as it ensures the logical qubits can process information accurately and reliably.
Think of fault-tolerant operations as a magician’s flawless performance. Regardless of the complexity of the tricks performed, the magician executes each one with precision, leaving no room for errors. Fault-tolerant gate implementations strive to provide a similar level of perfection, enabling logical qubits to carry out reliable and fault-tolerant quantum computations.
The Future of Advancements in Dynamically Generated Logical Qubits
As advancements in dynamically generated logical qubits continue to unfold, the future of quantum computing holds immense promise. These advancements will not only enhance the performance and reliability of quantum computers but also enable the development of practical quantum algorithms and applications.
With each milestone achieved, we move closer to a future where quantum computers will revolutionize industries, solve complex optimization problems, and contribute to scientific breakthroughs.
It is essential to foster collaboration and knowledge-sharing in this rapidly evolving field. By working together, researchers and scientists can accelerate the progress in dynamically generated logical qubits and overcome the remaining challenges.
As the quantum computing ecosystem expands, we can look forward to groundbreaking innovations and a quantum revolution that will shape the future of technology.
Frequently Asked Questions
In this section, we will address some common questions about advancements in dynamically generated logical qubits.
How are dynamically generated logical qubits different from traditional qubits?
Traditional qubits are susceptible to noise and errors, which can negatively impact the accuracy of quantum computations.
Dynamically generated logical qubits, on the other hand, are designed to be more resilient to errors. They use error correction techniques and sophisticated algorithms to mitigate the effects of noise, allowing for more reliable quantum computations.
Dynamically generated logical qubits also have the advantage of being able to perform more complex calculations than traditional qubits.
This is due to their ability to store and process information in a more efficient and robust manner, opening up new possibilities for quantum computing applications.
What are the main advancements in dynamically generated logical qubits?
One of the key advancements in dynamically generated logical qubits is the improved error correction codes used to protect the qubits from noise and errors.
These codes are designed to detect and correct errors, ensuring the accuracy of quantum computations. Researchers have made significant progress in developing more efficient and powerful error correction codes for logical qubits, enhancing their overall performance.
Another important advancement is the development of novel algorithms specifically designed for dynamically generated logical qubits.
These algorithms exploit the unique properties of logical qubits to perform complex computations more efficiently. As a result, researchers are able to tackle more challenging problems and speed up quantum simulations and optimizations.
What impact do advancements in dynamically generated logical qubits have on quantum computing?
The advancements in dynamically generated logical qubits have a significant impact on the field of quantum computing. They enable more reliable and accurate quantum computations by reducing the effects of noise and errors, leading to better results.
This opens up new possibilities for solving complex problems that were previously beyond the reach of traditional quantum computing.
Furthermore, these advancements pave the way for practical applications of quantum computing in various fields, such as cryptography, optimization, and drug discovery.
With the increased stability and computational power of dynamically generated logical qubits, researchers and companies can explore new avenues for solving real-world problems and accelerating scientific discoveries.
Are there any challenges associated with the advancements in dynamically generated logical qubits?
While there have been significant advancements in the field of dynamically generated logical qubits, there are still challenges that need to be addressed.
One of the main challenges is the requirement for large-scale, error-corrected quantum systems. Currently, the implementation of dynamically generated logical qubits is limited to small-scale systems due to the technical difficulties in scaling up quantum processors.
Another challenge is the integration of dynamically generated logical qubits with existing classical computing infrastructure.
As quantum computing continues to evolve, researchers need to develop efficient methods for interfacing with classical computers, enabling seamless integration and maximizing the benefits of both classical and quantum computing.
What does the future hold for dynamically generated logical qubits?
The future of dynamically generated logical qubits is promising. Researchers are actively working on overcoming the existing challenges and advancing the field even further.
We can expect to see continued progress in error correction techniques, algorithms, and hardware implementations, leading to more powerful and reliable dynamically generated logical qubits.
With these advancements, we can envision a future where quantum computers with dynamically generated logical qubits are widely available, offering unprecedented computational capabilities.
This can revolutionize industries ranging from finance to healthcare, unlocking new opportunities and transforming the way we solve complex problems.
Scientists are making exciting breakthroughs in creating more powerful and efficient quantum computers. One key development is the advancement in dynamically generated logical qubits.
These logical qubits are like the building blocks of quantum computers and are responsible for storing and processing quantum information.
By using a technique called error correction, researchers are able to create stable logical qubits that are less prone to errors. This is a major step forward in the field of quantum computing, as it allows for the creation of larger and more reliable quantum systems.
With these advancements, scientists are optimistic about the future of quantum computing, and the many possibilities it holds for solving complex problems and revolutionizing industries such as healthcare, finance, and cryptography.