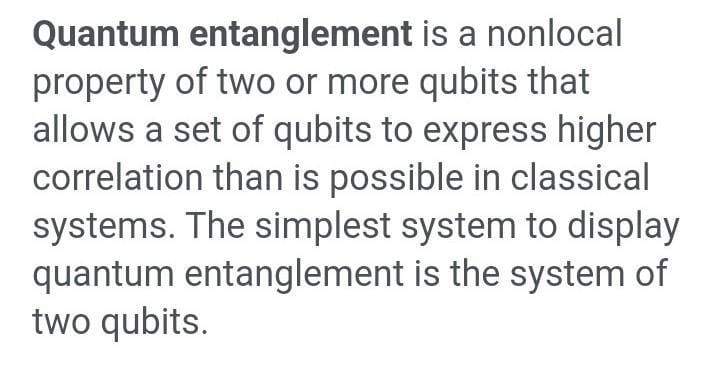
Hey there! I’m here to talk about a fascinating topic: the strong information correlation between qubits. You might be wondering, “Which term refers to this phenomenon?” Well, let’s dive in and find out!
You see, qubits are the building blocks of quantum computing, and they have this amazing ability to be in multiple states simultaneously. But there’s a special term we use to describe the strong information correlation between qubits. Can you guess what it is? Don’t worry if you can’t, we’re about to reveal the answer.
So, drumroll please… the term that refers to the strong information correlation between qubits is… “entanglement!” Yes, entanglement is what makes quantum computing so mind-blowing and powerful. Let’s explore more about this mind-bending concept together!
Shall we embark on this journey into the fascinating world of entanglement? Hold on tight, because we’re about to unravel the mysteries of this incredible phenomenon and discover its implications for the future of technology. Let’s get started!
Which Term Refers to the Strong Information Correlation Between Qubits?
When it comes to the strong information correlation between qubits, the term “entanglement” is commonly used. Entanglement refers to a quantum phenomenon where two or more qubits become interconnected, even when physically separated.
This correlation allows for the encoding and transmission of information in quantum systems. Entanglement plays a crucial role in various quantum computing and communication applications, making it a key concept in the field of quantum physics.
The Bell State (Capturing Quantum Correlation Between Qubits)
In quantum computing, the Bell state or the EPR state (named after physicists John Bell and Albert Einstein, Boris Podolsky, and Nathan Rosen, who proposed the EPR paradox) is the term that refers to the strong information correlation between qubits. The Bell state represents entanglement between two qubits, where their states are inseparable and dependent on each other.
The Bell state is described by four unique states, commonly known as the Bell basis:
- |Φ⁺⟩ = 1/√2(|0⟩⊗|0⟩ + |1⟩⊗|1⟩)
- |Φ⁻⟩ = 1/√2(|0⟩⊗|0⟩ – |1⟩⊗|1⟩)
- |Ψ⁺⟩ = 1/√2(|0⟩⊗|1⟩ + |1⟩⊗|0⟩)
- |Ψ⁻⟩ = 1/√2(|0⟩⊗|1⟩ – |1⟩⊗|0⟩)
Each of these states represents a specific quantum entangled configuration, where measuring the state of one qubit instantaneously determines the state of the other qubit, regardless of the distance between them. The Bell states play a crucial role in quantum teleportation, quantum cryptography, and other applications of quantum information theory.
Superposition and Entanglement (Foundation of Quantum Correlation)
To understand the term that describes the strong information correlation between qubits, it is essential to delve into the concepts of superposition and entanglement. Superposition refers to a qubit’s ability to be in multiple states simultaneously, thanks to quantum phenomena such as interference and wave-particle duality.
Entanglement, on the other hand, is a phenomenon that occurs when two or more qubits become linked in such a way that the state of one qubit is directly related to the state of the other qubits. In other words, entangled qubits share a strong correlation and cannot be described independently of each other.
This entanglement is what gives rise to the term that describes the strong information correlation between qubits. The Bell states, as mentioned earlier, are the prime example of entanglement, representing the highest level of correlation between qubits.
Applications and Implications of Quantum Correlation
The understanding and utilization of the strong information correlation between qubits have numerous applications and implications in the field of quantum computing and quantum information processing. Some of the key areas where this phenomenon plays a crucial role include:
- Quantum Teleportation: The Bell states and entanglement enable the teleportation of quantum information from one qubit to another, without physically transferring the qubit itself. This has implications in secure communication and quantum networking.
- Quantum Cryptography: The strong correlation between entangled qubits allows for secure communication channels that are inherently immune to eavesdropping or hacking attempts. This makes quantum cryptography a potential solution for unbreakable encryption.
- Quantum Simulation: Many real-world problems, such as chemical reactions or complex physical systems, are challenging to simulate using classical computers. Quantum correlation between qubits offers the potential to simulate these systems more efficiently and accurately.
The term that refers to the strong information correlation between qubits is vital in understanding and harnessing the power of quantum computing. As researchers and scientists continue to explore and innovate in this field, new breakthroughs and applications are expected to emerge, unlocking a world of possibilities for information processing and technological advancement.
Quantum Entanglement: The Foundation of Strong Information Correlation
Quantum entanglement is a fascinating phenomenon that gives rise to the strong information correlation between qubits. When two or more qubits become entangled, their states become intrinsically linked, exhibiting a strong correlation that cannot be explained by classical physics.
This entanglement forms the foundation of quantum computing and plays a vital role in various applications such as quantum teleportation and quantum cryptography. In this section, we will delve deeper into the concept of quantum entanglement and its implications.
Basics of Quantum Entanglement
Quantum entanglement occurs when the quantum states of two or more particles, such as qubits, become intertwined and interdependent. These particles can be separated by any distance but remain connected through a phenomenon known as non-locality.
One of the key characteristics of quantum entanglement is that measuring the state of one entangled particle instantly affects the state of the other particle, regardless of the physical distance between them.
This instantaneous correlation between entangled particles violates the principles of classical physics, where information transmission is limited by the speed of light.
Quantum entanglement is a delicate and fragile state that can be easily disrupted by interactions with the environment, making it challenging to preserve entanglement over prolonged periods. However, advances in quantum technology and quantum error correction techniques aim to overcome these challenges and enhance the stability of entangled states.
Applications of Quantum Entanglement
Quantum entanglement has far-reaching implications across various fields of science and technology. Here are some of the key applications of quantum entanglement:
Quantum Teleportation
Quantum entanglement is at the heart of quantum teleportation, a process that allows the transfer of quantum information from one qubit to another without physically moving the qubit itself. By entangling two qubits and performing specific operations, it is possible to teleport the quantum state from one qubit to another, regardless of the physical separation between them.
This process relies on the strong information correlation between the entangled qubits and has implications for secure communication and quantum networking.
Quantum Cryptography
Quantum entanglement is a crucial component of quantum cryptography, a field that aims to secure information transmission and ensure unbreakable encryption.
The strong correlation between entangled qubits enables the creation of secure communication channels that are inherently immune to eavesdropping. Any attempt to intercept or measure the entangled qubits would disrupt the entanglement, alerting the sender and ensuring the security of the transmitted information.
Quantum Computing
Quantum entanglement is the foundation of quantum computing, allowing for the creation of superposition and quantum gates that perform complex computations on multiple qubits simultaneously.
By harnessing the power of entanglement, quantum computers have the potential to solve computational problems that are currently infeasible for classical computers, offering advancements in fields such as optimization, machine learning, and cryptography.
Overall, quantum entanglement plays a pivotal role in the strong information correlation between qubits and serves as the backbone of quantum computing applications. Continued research and development in this field are expected to unlock new possibilities and pave the way for technological advancements in various domains.
Frequently Asked Questions
Welcome to our Frequently Asked Questions section, where we answer some common queries related to the strong information correlation between qubits.
What is the strong information correlation between qubits?
The strong information correlation between qubits refers to the tight connection between multiple qubits in a quantum system. In quantum computing, qubits can exist in a superposition of states, meaning they can represent multiple values simultaneously.
This correlation allows qubits to share and process information more efficiently than classical bits in traditional computing systems.
By entangling qubits, the strong information correlation between them enables quantum computers to perform complex computations and solve problems that would be challenging for classical computers.
As the number of qubits grows, this correlation becomes even more powerful, offering the potential for quantum computers to perform calculations and simulations that are currently impossible with classical machines.
How is the strong information correlation between qubits achieved?
The strong information correlation between qubits is achieved through a process called entanglement. Entanglement involves linking the quantum states of two or more qubits in such a way that the state of one qubit is dependent on the state of the others.
When qubits are entangled, the measurement of one qubit instantaneously affects the states of the other entangled qubits, regardless of the physical distance between them.
Entanglement is a fundamental property of quantum mechanics and is crucial for harnessing the power of quantum computing.
By entangling qubits, scientists and engineers can create complex quantum circuits and algorithms that take advantage of the strong information correlation between qubits, enabling quantum computers to perform calculations and solve problems more efficiently than classical computers.
What are the implications of the strong information correlation between qubits?
The strong information correlation between qubits has significant implications for various fields, including cryptography, optimization problems, and simulation of quantum systems. In terms of cryptography, quantum computers could potentially break many of the current cryptographic algorithms, as they can quickly search through a large number of possibilities due to their strong information correlation.
Furthermore, the strong information correlation between qubits allows quantum computers to more efficiently solve optimization problems, such as finding the best route for delivery trucks or optimizing supply chains.
Additionally, quantum simulation could benefit from the strong information correlation, enabling scientists to simulate systems that are not feasible to simulate with classical computers, such as chemical reactions or the behavior of complex molecules.
Are there any challenges in leveraging the strong information correlation between qubits?
Yes, there are several challenges in leveraging the strong information correlation between qubits. One major challenge is maintaining the coherence of qubits, as they are extremely sensitive to external disturbances and lose their delicate quantum properties through a process known as decoherence.
Protecting qubits from environmental factors that can disrupt their state is crucial for maintaining a strong information correlation and enabling quantum computers to function properly.
Another challenge is scaling up the number of qubits while preserving the strong information correlation. As the number of entangled qubits increases, so does the complexity of controlling and maintaining their entanglement.
Overcoming these challenges requires advancements in quantum hardware, error correction techniques, and developing new algorithms that can harness the power of large-scale quantum systems effectively.
How does the strong information correlation between qubits differ from classical computing?
The strong information correlation between qubits differs from classical computing in several ways. In classical computing, bits can only represent two states: 0 or 1. However, qubits can exist in superpositions of states, representing all possible combinations of 0 and 1 simultaneously.
This allows qubits to process and store a vast amount of information simultaneously, leading to exponentially increased processing power compared to classical bits.
Additionally, classical bits are independent of each other, whereas qubits are entangled, and their states are dependent on each other. This correlation enables qubits to perform complex computations and process information more efficiently than classical bits.
The strong information correlation between qubits is a fundamental property of quantum mechanics and is what gives quantum computers their unique computational power.
So, we’ve been talking about qubits and the correlation between them. Qubits are the building blocks of quantum computers, and they can hold both 0 and 1 at the same time. When qubits are in this “entangled” state, they can share information instantly, no matter how far apart they are.
This is called “quantum entanglement.” It’s like having a secret language that only qubits can understand, and they can speak to each other super-fast.
But there’s a twist! When we want to measure the information in one qubit, it “collapses” into a specific state, breaking the entanglement. This means we can’t continue to share information between qubits. So, when we talk about the strong correlation between qubits, we call it “quantum entanglement.”
It’s a mind-boggling concept that scientists are still trying to fully understand, but it’s the key to the power of quantum computers.